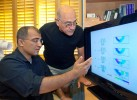
Through a combination of remote instrumentation, JPEG-style image compression algorithms and other key enhancements, Alexander Pines and members of his research group have been able to use Nuclear Magnetic Resonance (NMR) spectroscopy and Magnetic Resonance Imaging (MRI) to image materials flowing through microfluidic “lab-on-a-chip” devices and zoom in on microscopic objects of particular interest with unprecedented spatial and time resolutions.
"What excites me most about this new methodology is the possibility of a mobile, chip-based NMR/MRI platform for microfluidic analysis. Who knows? This might turn out to be useful for chemistry and biomedicine," says Pines. This latest work, which focused on MRI, has been reported in Science (doi: 10.1126/science.1192313) in a paper titled “Zooming in on Microscopic Flow by Remotely Detected MRI”.
Says Bajaj, first author on the Science paper, “We have been able to conclusively demonstrate the ability to record microscopic images of flowing macroscopic objects without loss of sensitivity, something that is impossible in conventional MRI. We were also able to illustrate how MRI can be used to measure flow dynamics quantitatively and with high spatial resolution in real microfluidic devices. The spatial resolution we achieved is sufficient to capture the results of hundreds or thousands of parallel assays on a microfluidic device. Furthermore, we recorded these images approximately one million times faster than could be done with a conventional MRI experiment. This means that experiments which would have taken years to complete, hence were impractical to consider, would now be possible.”
“Our goal is to develop NMR/MRI appliances for portable chemical analysis of complex mixtures, including blood, urine, and saliva,” Bajaj says. “Ultimately, we would like to make it possible to use NMR/MRI in point of care clinical analysis.”
In their new Science paper, Pines and Bajaj and their co-authors describe how they were able to apply MRI technology to studies involving microscopic flow through microfluidic or biological channels, or through porous materials. The key was the integration of several new elements into their remote NMR/MRI configuration. This included the fabrication of microsolenoid MRI probes with demountable microfluidic device holders, the design of remote MRI sequences for spatial encoding in the presence of motion, as well as for velocimetric measurements, and the use of JPEG-style compressive sampling algorithms for accelerated image encoding.
“The combination of remote NMR/MRI methods with these new elements spectroscopically mimics the implantation of a coil around a microscopic feature of interest and allows us to zoom in on the microscopic details of microfluidic flow dynamics in three spatial dimensions,” says Bajaj.
“The mechanism of remote detection is analogous to that of a magnetic recording tape on which complex data are first encoded and later read out by a single stationary detector as the tape advances.”